The Future of GMO Crops: a summary of the application of this technology
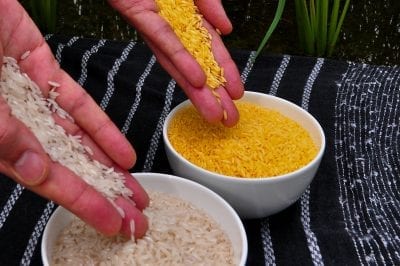
The USDA estimates that of the 430 million acres of cultivated crop land in the US, approximately 40% (170 million acres) is currently used to produce GMO crops. The current lines of GMO crops used in the United States have been primarily developed to aid farmers. These GMO crops either have lower production costs (weed control from herbicide application rather than repeated cultivations thus reducing labor costs) or can be expected to have higher yields under various stresses such as predation by specific insects. The targets of this technology have also primarily been major (large acreage) crops such as maize (corn), soybeans, cotton, and canola; however as of 2015, 14 GMO crops were in commercial production. The biotechnology companies that have developed these GMO products are able to reap financial benefits from their investments in this technology by selling seed to farmers. Farmers choose to purchase the seed because of the aforementioned commercial advantages. The consumer public does not consistently discern the financial advantages of the use of GMO seed by farmers because the cost of food includes many other factors besides the farm-based production cost. This business plan, or paradigm is referred to as the ‘first’ generation of GMO crops.
The expression of insect resistance (specifically to species of the beetle and moth genera of crop predator insects) has reduced the overall application of pesticides to US cropland. However, the reliance of the farm industry on GMO crops with herbicide resistance has increased the use of herbicides in agriculture. Overall, the adoption of first generation GMO crops has led to a slight (6% in one study) increase in agrichemical use overall. However, it is germane to note that, as far as impacts on human health (of both consumers and agricultural workers), different classes of chemicals have very different toxicities. Many chemicals used to control insect pests affect fundamental aspects of nerve cell action that are shared by insects and mammals including humans. On the other hand, herbicides typically control weed growth through the impairment of mechanisms unique to plant metabolism and thus are substantially less toxic to animals than many classes of insect poisons (pesticides).
An expansion or modification of these first generation GMO crop plants is now underway. Biotechnology companies are developing crops that have ‘stacked’ traits (referring to the transformation of target crops with multiple genes). In some cases, herbicide and insect resistance are expressed in the same GMO line, offering farmers the option of using crop lines that combine these traits. This is one type of ‘stacking’. However, stacking has also been used to address the issue of genetic resistance of weeds and insect predators. The wide adoption of GMO crops with insect and weed resistance in the US has inevitably led to the development of genetic resistance by the target organisms; weeds and insect predators. Biotechnology companies are developing GMO lines that express, for example, a number of different variations in the gene that encodes the protein that attacks insects feeding on the GMO plant. This could be predicted to delay the development of genetic resistance by the insects, but does not address the fundamental issue of sustainability underlying the problem.
The traits being introduced into the next, or ‘second’ generation of GMO crops plants include improvements and modifications in quality factors of the crop plants. This represents a shift in focus from the initial goals of the biotechnology industry. These quality factors include changes/improvements in the nutrition provided by the harvested portion of the crop plant. In addition, the goals of the biotechnology industry have included attempts to deal with complex traits (i.e. that are influenced by multiple genes) that impact the ability of the crop plant to efficiently use production inputs. These traits include drought tolerance (introduced into corn in 2011) and nitrogen-use efficiency. As of 2017, we are beginning to see commercial releases of GMO crop lines that display some of these second generation traits. An alfalfa variety has been developed with this technology that has reduced lignin and thus improved digestibility for animals. Disease resistance of papaya was one of the first applications of GMO technology several decades ago (although the acreage is small). However, application of GMO technology to improve resistance of crop plants to plant pathogens that are major diseases has begun. The list of crop plants with engineered resistance to viruses is expanding, and includes potato, plum, and squash. The USDA recently approved several important commercial varieties of potato that carry a gene from a wild species related to cultivated potato that has increased resistance to the fungal disease late blight- well known since the European potato famine of the late 19th century. It can be expected that both the range of crop plant species engineered for disease resistance, as well as the acreage dedicated to production of these crops will increase in the future. In addition, recent releases of GMO crop plants include alterations in the oil profile of canola and soybean to reduce trans-fat in food products (generated during processing), and enhanced levels of omega-3 fatty acid (in the case of soybean). Non-browning apples and potatoes (reducing shipping losses) have been commercialized recently. One of the largest processors of potatoes in the US developed the non-browning potato; a new paradigm in the US GMO crop industry.
Crop plants can also be used as ‘biofactories’ to produce important pharmaceuticals. This, and other innovative application of genetic engineering to plants are considered by some as a third generation of GMO technology development. It is unclear whether and to what extent this application of genetic engineering will be adopted. There have been some successes as well as failures on this innovative front of biotechnology. In one case, the production of human proteins in plants by a pharmaceutical company was not properly monitored and contained. The problem was not an issue of human health risk but rather a company not following agreed-upon protocols for monitoring, containment, and reporting of problems. On the other hand, the experimental application of a monoclonal antibody produced in plants was part of the (relatively unknown) story of saving infected physicians from the Ebola virus. Enhancing the ability of people in underdeveloped countries to have increased vitamin A precursor in their diet though the development of GMO crops has been demonstrated. In this case, ‘Golden Rice’ engineered to produce this nutrient could reduce the impact of this vitamin deficiency on human health; reducing blindness and death in (some estimates suggest one million per year of) people suffering from vitamin A deficiency. These innovative applications of plant GMO use certainly could be an area of future development.
Note: The National Academies of Science, Engineering and Medicine 2016 report ‘Genetically Engineered Crops: Experiences and Prospects was used as reference material for much of the information in this report.
By Gerald Berkowitz, Professor, UConn Department of Plant Science and Landscape Architecture
Published October 3, 2017